Engineering the low-carbon, cruelty-free, lab-grown hamburger of the future
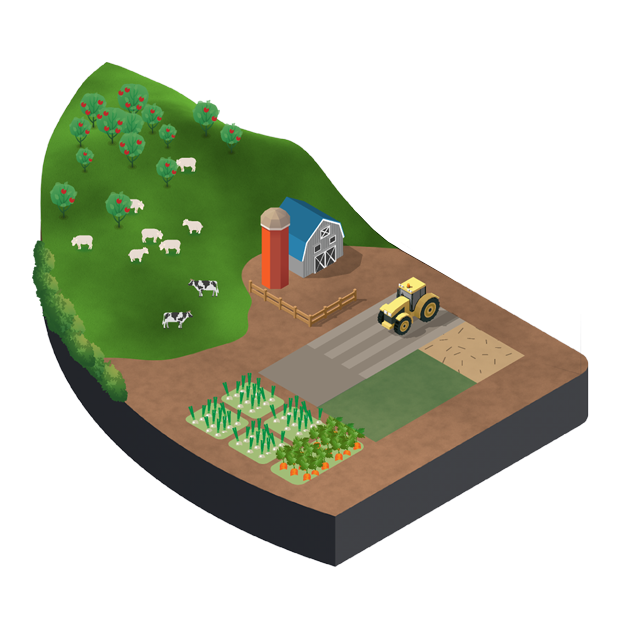
Like most people, I enjoy chomping down on an occasional hamburger. Not just the fast-food variety, but quite often the posh restaurant version. In fact, it is usually my go-to menu choice when eating with friends. (Particularly those who might judge me during the bill splitting process if I order a steak)!
But I am increasingly, and reluctantly, struggling with the ethics of meat-eating. Animal welfare in particular does not worry me. Like most meat-eaters, I am rather adept at turning a blind eye to that. My concern is about the environmental impact of rearing enough animals to feed a rapidly growing population of almost 8 billion people. There are about 1.5 billion cattle in the world, about one cow for every five people alive today. The problem is that those cows produce a lot of methane. In global warming terms – the equivalent of about 2 billion tonnes of carbon dioxide. That’s 4% of all greenhouse gas emissions from the dairy from cattle belching, flatulence and manure alone. Some estimates claim that the dairy and beef industries are responsible for about 14.5% of all greenhouse gas emissions.
The carbon cost of a cheeseburger
I wanted to illustrate this problem in the context of a takeaway burger meal. I know that this isn’t everyday food for many people, but it illustrates the problem of agriculture-based greenhouse gas emissions in a context that most people are familiar with. And then, we’ll talk about how we can (with the help of engineering simulation) significantly reduce those emissions.
These estimates come from the fantastic book “How Bad Are Bananas? The Carbon Footprint of Everything”, which I highly recommend. Mike Berners-Lee goes to extraordinary lengths to calculate the carbon impact of everyday items. His estimates include the carbon cost of growing and rearing the ingredients of the meal. This includes the cost of getting them to your plate, including processing, transportation, packaging and refrigeration. But not of cooking the meal, which depends on how “green” your energy supply is.
The results are staggering: about 87% of the greenhouse gas emissions for a hamburger come from the beef patty, which accounts for 2.84kg of CO₂e. The other ingredients contribute just 400g. Most of which is due to the cheese (obviously also a product of cow farming).
Would you like to make a meal of that?
Since most of us order our burgers as part of a “meal”, I’ve also calculated the carbon cost of adding to the order. Once again, the most significant contribution comes from dairy milkshake that adds another kilogram of emissions to the total.
Estimates based on “How Bad Are Bananas? the Carbon Footprint or Everything”, Mike Berners-Lee, 2020, Profile Books, ISBN: 9781788163811 and “Food and Climate Change Without the Hot Air”, Sarah Bridle, UIT Cambridge Ltd. Does not include green house gas emissions due to the cooking process.
The true cost of what you eat
Think that eating a banana that has likely traveled halfway around the world to get to your breakfast table is worse for the environment than a locally sourced beef burger with cheese? Think again!
The immediate solution
Most of us need to eat fewer animal products. The total carbon footprint of a cheeseburger, fries and milkshake is about 4.4kg of greenhouse gas. By replacing the beef patty with a bean burger (about 630g of CO₂e) and choosing a soda instead of the dairy milkshake, the quantity of greenhouse gases emitted decreases to about 1.1kg of CO₂e.
Many vegetarian or vegan readers of this article would argue that the ideal solution would be for everyone to immediately stop eating meat (and perhaps other animal proteins) immediately and forever. However, I’m not convinced that this is a pragmatic solution to the climate emergency. A recent study calculated that 14% of consumers are vegetarian (11%) or vegan (3%). According to the study, another 20% of consumers are “flexitarians” who limit their meat intake. (I’d probably count myself among them). It seems unlikely to convince many consumers to entirely turn their back on meat in the timescales required to avert a climate disaster.
However, it should be abundantly clear that our planet cannot cope with the estimated 70 billion farm animals required to feed a rapidly growing population of almost 8 billion humans.
Green house gas emissions per 1000 kilocalories
Greenhouse gas emissions are measured in kilograms of carbon dioxide equivalents (KgCO₂eq) per 1000 kilocalories. This means non-CO₂ greenhouse gases are included and weighted by their relative warming impact.
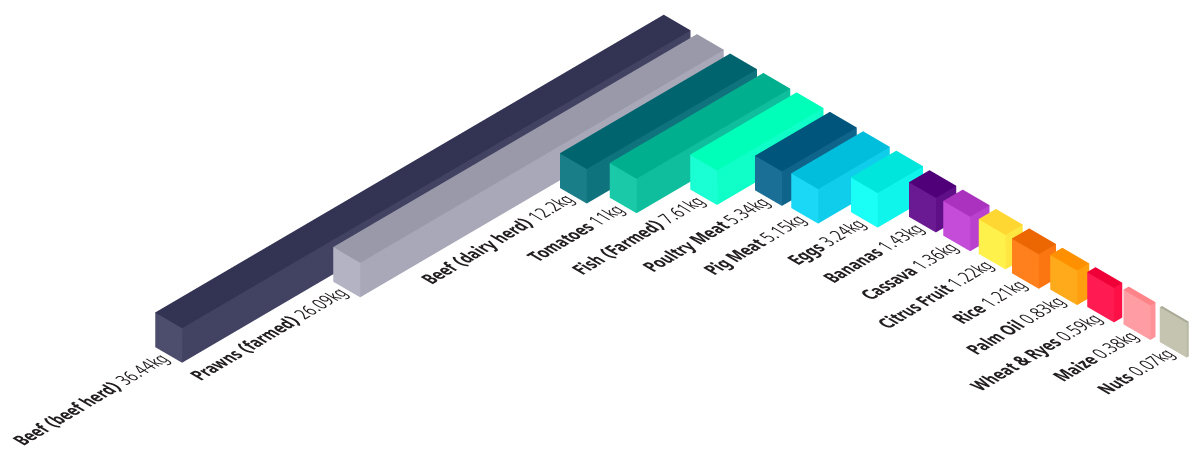
Source: Poore, J., & Nemecek, T. (2018). Additional calculations by Our World in Data. Note: Data represents the global average greenhouse gas emissions of food products based on a large meta-analysis of food production covering 38,700 commercially viable farms in 119 countries. Our WorldInData.org/environmental-impacts-of-food • CC BY
Engineering a solution: lab-grown meat
From a Climate Emergency perspective, the major problem of farming livestock for food is that the living breathing farm animals produce copious amounts of methane – an incredibly potent greenhouse gas – through belching, flatulence and manure. This is a particular problem for ruminants such as cows and sheep, but true of all farm animals. Almost all animal products have much higher greenhouse gas emissions than vegetables per calorie of food produced.
In December 1931, British Prime Minister Winston Churchill wrote an article in which he tried to predict the state of the world “50 years hence”. Churchill wrote: “We shall escape the absurdity of growing a whole chicken to eat the breast or wing by growing these parts separately under a suitable medium.”
He had a point. An enormous amount of time, energy and natural resources go into breeding, feeding and processing animals for meat production. As a species, we grow enough crops to feed more than 10 billion people. Of this total, around 2 billion people’s worth of food is fed to farm animals.
And as predictions go, it was reasonably accurate, although Churchill was a little optimistic regarding time scales. The world’s first lab-grown meat hamburger was served to food critics in London in 2013 (82 years after his prediction). One food critic described the burger: “This is meat to me. It’s not falling apart. The mouthfeel is like meat. I miss the fat, there’s a leanness to it, but the general bite feels like a hamburger.”
That burger was grown from stem cells extracted through biopsy from the muscles of a living cow. When placed in a petri-dish full of a nutrient-rich medium, under carefully controlled conditions, those cells begin to divide. And then divide again, and again, until there are thousands of cells, or at least enough to build a hamburger. (Apparently, it takes about 20,000 muscle cells to make a burger).
Mass produced lab-grown meat
Despite this considerable achievement, nine years have passed since the advent of the “lab-grown burger”. But we’ve yet to see lab-grown cruelty-free low carbon meat in our grocery stores (or our favorite takeaway burger joint). Why is that?
Part of the problem is regulatory approval for the consumption of lab-grown meat from food licensing agencies. Although that hurdle has recently been overcome in at least one territory. Another issue is consumer acceptance. Are the meat-eating public prepared to eat meat grown in Petri dishes rather than on farm animals? I’ll try to address that question at the end of this article.
The real challenge for producers of lab-grown meat is creating thousands of kilograms of biomass (given that a single head of cattle can produce around 450kg of beef) from a few stem cells. For commercial production, these cells are grown inside bioreactors, carefully controlled nutrient-enriched mixing environments in which the temperature, oxygen, carbon dioxide and pH are precisely controlled to maximize the yield of cells. If that environment is slightly wrong, the cells will fail to divide, divide into the wrong type of cells, or possibly die. For this reason, the contents of the bioreactor’ must be constantly mixed.
Unfortunately, mammalian cells are also susceptible to the shear stress caused by the mixing process. In particular, the shearing action of turbulent vortices can detach the cells from the scaffold on which they grow.
Therein lies the challenge – biological processes do not scale linearly with the geometric size of the bioreactor. Maintaining the ideal cultivation conditions developed in a 10-litre bench experiment in a 4,000-litre industrial bioreactor is a difficult engineering problem. The design of an industrial-scale bioreactor will be significantly different from that of the laboratory-scale bioreactors in which the original process was designed.
Designing larger vessels in which optimal cell growing conditions can be adequately maintained is almost entirely dependent on computational fluid dynamics. Although cultured meat producers are understandably being secretive about their commercially sensitive processes, there are many publicly available examples from the medical industry on how Simcenter is being used to grow human cells for transplants, monkey kidney cells for vaccine production, or bioreactor impeller design.
How can Simcenter help?
A few years ago, we talked to ABEC, manufacturers of the world’s largest bioreactors. We discussed how they use Simcenter to scale the production of microorganisms. Although our conversations were about cultivating cell colonies for biopharmaceutical purposes rather than meat production, the principles described are the same.
Paul Kubera, vice president of process technology at ABEC, told us,
“A typical scenario might involve a project that has moved from the laboratory bench at the tens-of-litres scale to process development, which may be operating on a few hundreds-of-litres scales. And then into production where they need to ramp up by thousands of litres in multiple units.”
The challenge with bioreactors is keeping the host-cell platform alive. According to Kubera,
“The growth of the organism must be supported – it needs food, a carbon source and to take in oxygen and give off carbon dioxide. It is critical to be able to deliver a known amount of oxygen in a given timeframe and remove carbon dioxide for all the organisms in the vessel.”
Kubera continued:
“With Simcenter, we can run a computational simulation of the laboratory configuration and confirm the same results. We can then run a large-scale simulation and be confident that the measured performance of the delivered equipment will track with expectations. As an example, we demonstrated that we can cut blend time 50% by using laboratory tests to screen options and Simcenter simulation to extend the results.”
Bioreactors can be highly efficient once you perfect the scaling process. Whereas it takes months or years to grow sufficient quantities of meat on a living animal, the same process can take just 2 to 4 weeks in a bioreactor. Since the meat is grown from stem cells, no animals are harmed in the process. The only real ingredients are water, chemical nutrients, and heat. (Rather than intensively cultivated grains that are used to feed most cattle). Most importantly of all, the process does not cause the release of large amounts of methane. Meaning that the greenhouse gas emissions should be more than 70% lower than for farm-grown meat.
Would you eat lab-grown meat?
Another potential problem is market adoption. Are consumers ready to eat meat grown in a petri-dish rather than on a farm? Are we prepared to separate the meat from the animal, as it were? To an extent, as consumers, we do this every time we visit the grocery store. We browse cabinets full of shrink-wrapped meat, which is often dyed pink to make it seem less flesh-like.
The whole meat-buying experience is deliberately sanitised to remove any connection to the animal’s death. Unlike in the old days of the butcher’s shop, you can’t even smell the meat in a grocery store. I wonder how many habitual meat-eaters would, if given the opportunity, visit an abattoir to witness how meat is actually “made”?
The truth is that most of us eat lots of things that we don’t know the origin of. For example, Quorn, a single cell mycoprotein derived from a fungus, is grown in bioreactors. Since it was launched in the late 1980s, Quorn has achieved a decent market penetration in Europe as a meat substitute, with manufacturers claiming that it has a carbon footprint “at least 80% less than that of beef”.
Because I’m trying to reduce my carbon footprint (and my meat consumption), I’ve recently started eating “plant-based burgers”. I have no idea what goes into them, but they taste OK, even if the texture is a bit soggy. Personally, I would happily eat a lab-grown meat burger given the opportunity. But of course, ground meat products like burgers only make up part of animal product consumption. In Singapore, the first lab-grown chicken meat is on sale. Elsewhere there are plans to produce lab-grown cow’s milk and lab-grown human breast milk.
How about a lab-grown steak?
The real challenge for me is the cultivation of a perfect lab-grown steak. Unlike burgers and chicken breast, which are homogeneous, the steak-eating experience depends on a rather heterogeneous distribution of protein and fat. However, even that might soon be possible using 3D-printing techniques.